Harnessing the Sun: The Marvels and Mechanics of Solar Panels
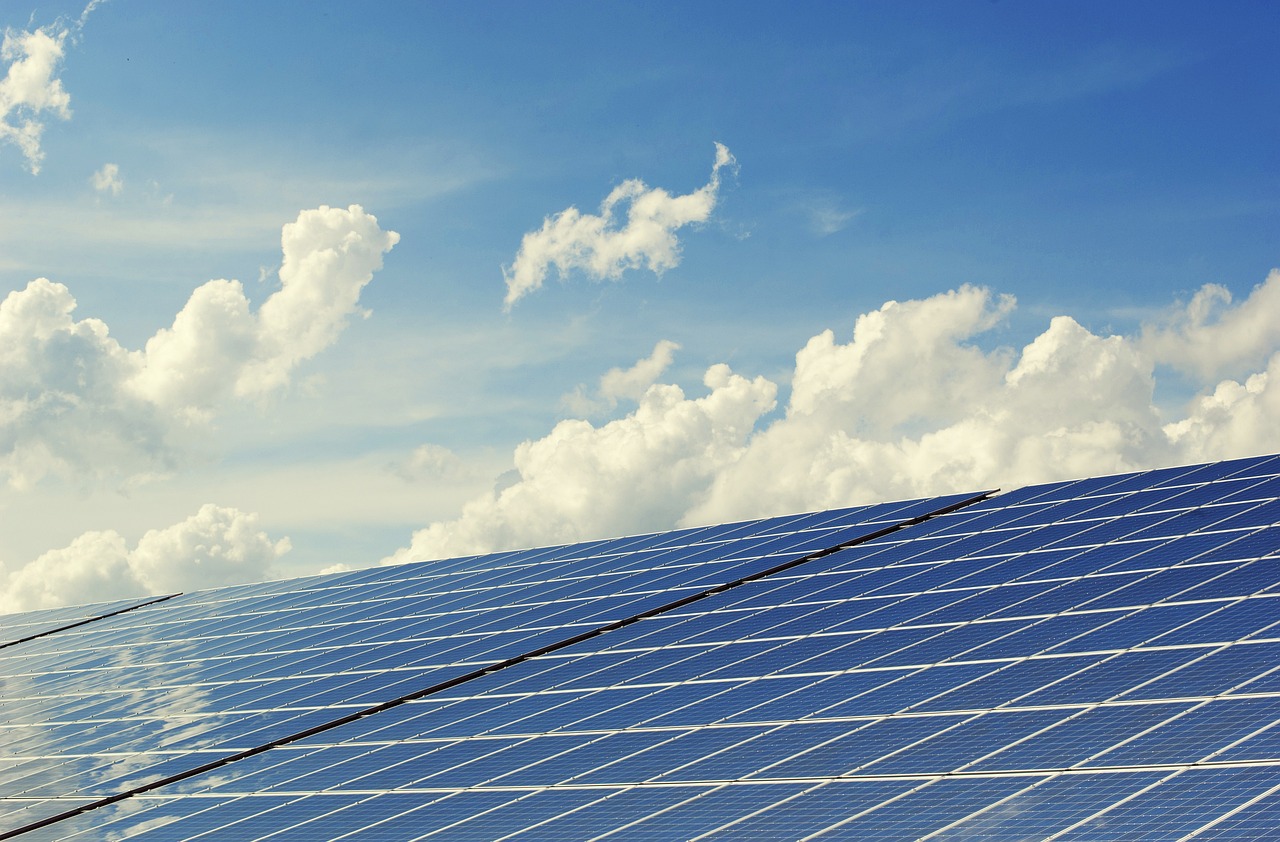
In the realm of sustainable energy, solar power emerges as a frontrunner, offering a beacon of hope amidst the challenges of climate change and energy security. Central to this solar revolution are solar panels, intricate devices that harness the sun&8217;s abundant energy and convert it into usable electricity. In this comprehensive exploration, we delve into the intricate mechanics, diverse applications, and promising future of solar panels.
The concept of converting sunlight into electricity dates back to the 19th century, with the discovery of the photovoltaic effect by French physicist Alexandre-Edmond Becquerel in 1839. However, it wasn&8217;t until the mid-20th century that practical applications of solar energy began to emerge, driven by advancements in semiconductor technology and space exploration.
In 1954, Bell Laboratories developed the first practical solar cell, ushering in a new era of solar technology. These early solar cells, made from crystalline silicon, paved the way for the modern solar panel. Over the decades, continuous research and innovation have led to significant improvements in efficiency, durability, and cost-effectiveness, making solar power an increasingly viable alternative to traditional fossil fuels.
The Mechanics of Solar Panels
At the core of every solar panel lies photovoltaic cells, commonly referred to as solar cells. These cells are typically made from semiconductor materials, such as silicon, which possess the unique property of converting sunlight directly into electricity through the photovoltaic effect. When sunlight strikes the surface of a solar cell, it dislodges electrons from the atoms within the semiconductor material, generating an electric current. This flow of electrons is then captured and harnessed through an electrical circuit, producing usable electricity.
Solar panels are composed of multiple interconnected solar cells, arranged in a grid-like pattern on a supportive substrate. These cells are typically housed within a protective enclosure, such as tempered glass, to shield them from environmental factors and ensure longevity. Electrical contacts are strategically placed on the surface of the solar panel to facilitate the flow of electricity generated by the solar cells.
Solar panels come in various forms, each tailored to specific applications and environmental conditions. The two most common types of solar panels are monocrystalline and polycrystalline.
Monocrystalline Solar Panels: Recognizable by their uniform dark color and rounded edges, monocrystalline panels are crafted from high-purity silicon ingots. These panels boast high efficiency rates and superior performance in low-light conditions, making them ideal for residential and commercial installations where space is limited.
Polycrystalline Solar Panels: Characterized by their blue speckled appearance, polycrystalline panels are manufactured using melted silicon fragments. While slightly less efficient than monocrystalline panels, polycrystalline panels offer a cost-effective alternative, making them well-suited for large-scale utility installations where space is abundant.
Despite their numerous advantages, solar panels face several challenges that warrant attention and innovation:
Intermittency and Energy Storage: Solar power generation is inherently intermittent, fluctuating with weather conditions and diurnal cycles. To address this challenge, advancements in energy storage technologies, such as batteries and pumped hydro storage, are crucial for storing excess solar energy and ensuring grid stability.
Land Use and Environmental Impact: Large-scale solar installations require significant land resources, raising concerns about habitat disruption, land degradation, and competing land-use priorities. Sustainable siting practices, environmental assessments, and land-use planning are essential to minimize adverse impacts and maximize co-benefits.
Material Resources and Recycling: The production of solar panels relies on finite resources, such as silicon, glass, and rare earth elements. To ensure the long-term sustainability of solar energy, efforts to develop alternative materials, improve recycling technologies, and minimize resource extraction and waste generation are imperative.
Policy and Regulatory Frameworks: Policy uncertainty, regulatory barriers, and market distortions hinder the widespread deployment of solar panels and impede the transition to a renewable energy future. Supportive policies, such as feed-in tariffs, net metering, renewable portfolio standards, and carbon pricing, are essential for incentivizing investment, fostering innovation, and leveling the playing field for solar energy.
Looking ahead, the future of solar panels appears promising, fueled by ongoing technological advancements, declining costs, and growing public and political support for renewable energy. With continued innovation, collaboration, and investment, solar panels will play a pivotal role in powering a cleaner, greener, and more sustainable world for generations to come.
Conclusion
Solar panels represent a remarkable marriage of science, engineering, and innovation, offering a tangible solution to the pressing challenges of climate change, energy security, and economic development. As we harness the boundless energy of the sun, solar panels illuminate the path towards a brighter, more sustainable future for all.
Tanbir Ahmed
Fsbbig